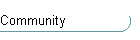
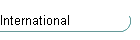
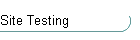
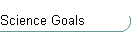
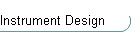
| |
Report of Galactic Structure Session
Session Leader: Bruce Elmegreen
Nuclear Region
Infrared line observations (C II, O I, CO) of the nuclear region of the
Milky Way (Lugten et al. 1986; Genzel et al. 1990; Poglitsch et al.
1991; Nakagawa et al. 1995) have shown a rich structure with clouds,
arched filaments associated with ratio continuum structures, and a small
tilted disk. The C II is probably at the interface between dense clouds
and bright uv sources, such as OB stars. Some of the material may be
accreting to the Sgr A core. Submillimeter continuum emission from cool
dust in this region shows sources associated with molecular clouds and a
ridge between them (Dent et al. 1993).
A ten meter sub-mm wave telescope at the South Pole can map the nuclear
region in C I emission at 370 and 600 microns, N II emission at 205 microns,
and in the high J lines of CO and other molecules. The C I map can be
compared with the N II map and a C II map from ISO to determine variations
in the PDRs and diffuse ionization structure. The brightness of a PDR
indicates the relative location of the neutral gas and the source of
ionization. This could be used to map the relative positions of the
dense clouds and uv sources in the nuclear region. The CO lines can be
used to give gas densities in nuclear molecular clouds. The densities
in these clouds are known to be very high, so sub-mm J transitions are
necessary to determine their values and the corresponding pressures.
Extensive polarization maps of the nuclear region and filaments at sub-mm
wavelengths will reveal the magnetic field orientation and structure
with unprecedented detail. Since much of the theoretical work on
nuclear gas processes and nuclear jets involves magnetic forcing, these
observations should stimulate future developments of the theory.
C I maps of the extended nuclear region will include the Galactic Bar and
possible evidence for streaming motions along the leading edge of the
bar, as observed in other galaxies.
Polarized Dust Emission from other Locations
Infrared polarization from aligned dust emission provides a rich source
of information on magnetic field orientation in a wide variety of
regions in the Galaxy (Hildebrand 1988; Barvainis, et al. 1988; Flett &
Murray 1991).
Extensive maps of polarized dust should be made at 350 microns for every
possible environment: in high latitude loops, dense clouds, suspected
shock fronts near supernovae remnants, continuous (C-type) shock fronts
in molecular clouds, diffuse clouds, connections between clouds, and so
on. At larger distances from the Sun, chimneys or vents of hot gas to
the halo might be found in regions where the polarization becomes
perpendicular to the Galactic plane.
Variations in the ratio of sub-mm polarization to continuum emission
should also be mapped to help understand the alignment of grains and how
it depends on gas density, temperature, and velocity field. Emission
line polarization is a new field. Many discoveries about the origin and
dynamics of Galactic magnetic fields and about dust alignment should
come from these studies.
Local Clouds
The intricate "fractal" structure of local and other clouds,
particularly at high latitude, was originally observed in warm dust
continuum emission by IRAS (Low et al. 1974). A similar structure is
inferred for unresolved molecular clouds because of the ease of
penetration by uv light, as indicated by pervasive emission from C II
(Stutzki et al. 1988; Howe et al. 1991; Jaffe et al. 1994; Boreiko &
Betz 1995) and other fine structure lines (Meixner 1992; Stacey et al.
1993). Penetrating C II has also been observed from diffuse clouds at
high Galactic latitude (Bock 1993), and from the rho Oph cloud (Yui
et al. 1993). Other evidence for fractal structure comes from
perimeter-area relations in CO or IRAS maps (Dickman, et al. 1990;
Falgarone et al. 1991), and from the cloud size distribution and the
mass-size relation (Elmegreen & Falgarone 1996).
Theoretical studies of interstellar gas dynamics and star formation
depend our knowledge of the detail structure of gas and its motions.
Most likely, the observed structure and motion is related to turbulence,
but it could also be affected by regular magnetic waves, localized
stellar or pre-main-sequence winds, and large scale flows, as in a
spiral wave.
The ten meter telescope will be the most important groundbased
instrument for mapping the detailed structure of neutral gas (C I;
Phillips & Huggins 1981) and dust emission in local and other Galactic
clouds. This should clarify the suspected fractal or filamentary
structure like no other experiment. The angular resolution will be much
higher than for IRAS or H I studies at 21 cm, and C I is pervasive for
dense diffuse gas (n > 300 cm-3). Comparisons between the continuum
or C I structures and the magnetic field orientation obtained with the
same telescope will give unprecedented clarity to many processes
relevant to interstellar gas dynamics and cloud formation.
From the South Pole, regions that can be studied include diffuse clouds
in the outer Galactic flare and warp, the Galactic center and Carina
spiral arm, the Gum nebula, eta Carina and other important nebulae and
molecular clouds, much of Lindblad's ring and local high latitude
clouds, and possibly the Magellanic stream and other high velocity
clouds. Studies using both continuum from dust and line emission from
C I and N II should be made.
The Galactic Plane
Diffuse emission from C II has been observed along the galactic plane
from photodissociation regions near and inside known molecular clouds
and possibly from the diffuse ionized medium (Shibai et al. 1991).
Emission from this and many other FIR fine structure lines, including C I
and N II, was observed by COBE (Wright et al. 1991). Similar emission
was mapped for the nearby galaxy NGC 6946 (Madden 1993).
The theory of diffuse line FIR emission was considered by Wolfire et al.
(1995), not including the C I.
The ten meter telescope should be used to survey the Southern Galactic
plane in C I and N II. The N II emission comes from H II regions and will
be a much better tracer of distant ionization than H alpha because
there is no dust extinction for N II. The C I comes from dense gas,
including both atomic and molecular regions. C I absorption against
background dust continuum might also be observed. The Southern molecular
ring in our Galaxy should be mapped in detail.
Comparison between C I and CO should reveal the extensive diffuse cloud
structure around molecular clouds, and the ratio of these two line
strengths should be a good measure of the molecular fraction, which is
known to vary with radius in the Galaxy. C I should also trace galactic
dynamics as well as CO, but it should also include slightly lower
density regions, thereby covering a larger volume filling factor than CO
or other molecules.
Stellar and Gaseous Distance Tracers
FIR emission from stars, whether from lines or the continuum, or from
variable or steady sources, may eventually be found to contain enough
information to determine the stellar distance independent of other
information, such as velocity. Such a discovery, like a period-
luminosity relation for Cepheids, would allow distance determinations in
the infrared, which has the advantage over optical methods of begin free
from dust extinction.
Possible sources for such a distance calibrator might be Carbon stars,
Carbon rich WR stars, LGB stars, or protostars. For example, one might
search for a correlation between intrinsic IR luminosity and IR spectral
linewidth, as might arise in a wind or from associated disk rotation, or
one might search for an IR period-luminosity relation in evolved stars.
If such a stellar IR distance indicator is found, then it might be
possible to map the far side of the galactic disk, completing the spiral
arcs that are found on the near side. IR distances to nearby galaxies
might be possible too, using bright stars that are rare enough to have
no similar stars in the same field of view.
A distance indicator might be present in the gas as well, using a size-
linewidth relation, for example. If IR fine structure lines are found
to show a linewidth correlation with the size of the mapped cloud, as is
the case for CO lines in nearby molecular clouds, then maps of the far-
side galactic plane at high spatial resolution (high enough to resolve
the scale height), can be decomposed into discrete clouds with
measurable linewidths and sizes. This should give the 3D structure of
the Milky Way when the observed sizes are converted into distances using
the intrinsic sizes from the linewidths. C I would seem to be a good
choice for such an emission map because of its clear association with
molecular and other neutral gas clouds. Preliminary calibrations on
local clouds would be necessary.
References
- Barvainis, R., Clemens, D.P., & Leach, R. 1988,
AJ, 95, 510
- Bock, J.J., Hristov, V.V., Kawada, M., Matsuhara, H.,
Matsumoto, T., Matsuura, S., Mauskopf, P.D.,
Richards, P.L., Tanaka, M., & Lange, A.E. 1993, ApJ, 410, L115
- Boreiko, R.T., & Betz, A.L. 1995, ApJ, 454, 307
- Dent, W.R.F., Matthews, H.E., Wade, R., &
Duncan, W.D. 1993, ApJ, 410, 650
- Dickman, R.L., Horvath, M.A., & Margulie, M. 1990, ApJ,
365, 586
- Elmegreen, B.G., & Falgarone, E. 1996, ApJ, 471, 816
- Falgarone, E., Phillips, T., & Walker, C.K. 1991, ApJ,
378, 186
- Flett, A.M., & Murray, A.G. 1991, MNRAS, 249, 4p
- Genzel, R., Stacey, G.J., Harris, A.I., Townes, C.H.,
Geis, N., Graf, U.U., Poglitsch, A., & Stutzki, J.
1990, ApJ, 356, 160
- Hildebrand, R.H. 1988, Q. Jl. R., Astr. Soc.,
29, 327
- Howe, J.E., Jaffe, D.T., Genzel, R., & Stacey, G.J.
1991, ApJ, 373, 158
- Jaffe, D.T., Zhou, S., Howe, J.E., Herrman, F., Madden,
S.C., Poglitsch, A., van der Werf, P.P., & Stacey, G.J.
1994, ApJ, 436, 203
- Low, F.J., et al. 1974, ApJ, 278, L19
- Lugten, J.B., Genzel, R., Crawford, M.K., & Townes, C.H.
1986, ApJ, 306, 691
- Madden, S.C., Geis, N., Genzel, R., Herrmann, F.,
- Nakagawa, T., Doi, Y., Yui, Y.Y., Okuda, H.,
Mochizuki, K., Shibai, H., Nishimura, T., & Low, F.J.
1995, ApJ, 455, L35
- Jackson, J., Poglitsch, A., Stacey, G.J., & Townes, C.H.
1993, ApJ, 407, 579
- Meixner, M., Haas, M.R., Teilens, A.G.G.M., Erickson,
E.F., & Werner, M. 1992, ApJ, 390, 499
- Phillips, T.G., & Huggins, P.J. 1981, ApJ, 251, 533
- Poglitsch, A., Stacey, G.J., Geis, N., Haggerty, M.,
Jackson, J., Rumitz, M., Genzel, R.,
& Townes, C.H. 1991, ApJ, 374, L33
- Shibai, H., et al. 1991, ApJ, 374, 522
- Stacey, G.J., Jaffe, D.T., Geis, N., Genzel, R.,
Harris, A.I., Poglitsch, A., Stutzki, J., & Townes, C.H. 1993,
ApJ, 404, 219
- Stutzki ,J., Stacey, G.J., Genzel, R., Harris, A.I., Jaffe,
D.T., & Lugten, J.B. 1988, ApJ, 332, 379
- Wolfire, M.G., Hollenbach, D., McKee, C.F.,
Tielens, A.G.G.M., & Bakes, E.L.O. 1995, ApJ, 443, 152
- Wright, E.L., et al. 1991, ApJ, 381, 200
- Yui, Y.Y., Nakagawa, T., Doi, Y., Okuda, H.,
Shibai, H., Nishimura, T., & Low, F.J. 1993, ApJ, 419, L37
|