Supernovae
Supernovae are simply stars which explode. It turns out there is more than one way for a
star to explode, and hence we have more than one type of supernovae. To understand supernovae,
we need to understand stars.
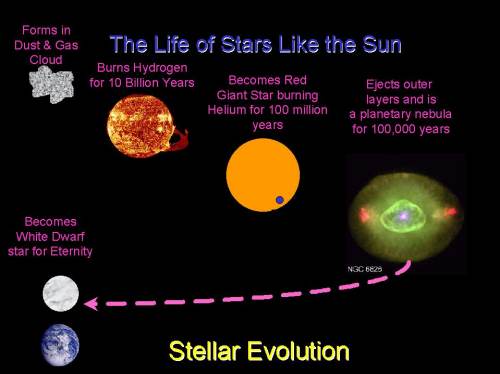
Our sun was formed out of clouds
of dust and gas. In these clouds, little clumps collapsed, and their gravity continued to
attract more and more material until the material heated up and became dense enough to start the
fusion of Hydrogen to Helium. A star spends most of its life converting its Hydrogen to
Helium - we think our sun will do this for a total of about 10 billion years. In case you are
worried, we have about another 5.5 billion years left before the sun goes into its next
stage, burning Helium to Carbon. In this stage our Sun will swell up hundreds of times its
current size, swallowing the Earth, and glowing hundreds of times brighter than it is now.
We call this phase of a star its red giant phase. The sun eventually runs out of Helium to
burn, and this is the end of the road for the Sun. Its core collapses into a very dense star
known as a white dwarf, and its outer layers are released to form a planetary nebula (one
of the most spectacular sights in the sky). The planetary nebula soon disperses, and the
white dwarf is left to cool for eternity, fading into oblivion.
Stars more than 10 times the mass of our sun have much more exciting lives. They form
in much the same way as our sun, but are a million times brighter, and subsequently burn
their Hydrogen much quicker than our sun. The good life does not last long (it doesn't
take an astrophysicist to realise that if you only have 10 times more fuel, but are using it
up a million times quicker, you will run out of steam 100,000 times sooner). These stars
then quickly turn to Helium to satisfy their energy needs and become Red Super Giant
stars, expanding out to the orbit of Mars in the process. The Helium soon runs out, but
these stars are able to continue to process additional elements as atomic fuel.
After Helium, these massive stars convert their Carbon to Oxygen, and then into
successively heavier elements. Each conversion gives less and less energy, and support
the star's appetite for fuel for less and less time. The last few days of the star's 10 million
year life are spent burning Silicon into Iron.
Although iron can be fused into heavier elements such as Cobalt and Nickel, these
reactions do not act as a nuclear furnace, but rather as a nuclear refrigerator.
The star, which was held up by the pressure caused by all the heat generated (Hot air in a
balloon has more pressure causing the air to expand making the air lighter than the cooler
outside air), suddenly begins to fall onto itself, with the inner part of the star collapsing to
a neutron star, and the outer parts of the star being thrown off and forming a.....
A Type II supernova to be precise.
The picture shows supernova 1987A before and after as caught by David Malin and the Anglo Australian
Observatory. SN 1987A was discovered on February 23, 1987, and although its light took
170,000 years to reach Earth, it became easily visible to the naked eye. It was the closest
and brightest Supernova as seen from the Earth in the past 350 years.
So where is the next supernova going to be in our galaxy. Well, we are not sure. One good
guess is the the Eta Carinae, one of the most massive stars in our galaxy. This star will
explode at some point, maybe tomorrow, maybe in 10,000 years, but it will only visible
to the inhabitants of the southern hemisphere. (Picture from Mike Bessell, MSSSO)
My favourite candidate for the next supernova is Betelgeuse in the constellation Orion. It
could also explode tomorrow or in 10,000 years. When it does go, it will be bright, about
like the moon when it is 1/2 full, But it will be a point of light rather than a disk, and will
be a spectacular sight in the sky for many months. (Picture courtesy of Mike Bessell, MSSSO>
One of the most spectacular objects in the sky must have been the Supernovae of 1054
observed by the Chinese. Today this explosion is seen as the expanding remains of the
star (we call it the Crab Nebula), and inside is a pulsar which is the rotating neutron star
shining a beacon of light towards the Earth many times a second.
Type Ia Supernovae
Type Ia Supernova are the explosions of white dwarfs. This is a pinnacle that only a few
stars like our sun are able to achieve. Unfortunately we are not sure exactly how these
events occur. We think they are related to white dwarf stars which are near another star in
a binary system.
Chandrasekhar, as part of his Nobel Prize in Physics demonstrated that white dwarf stars,
if they become more massive than 1.4 times our sun can explode. They do this because at
this point, the forces (electrons repelling electrons) which keep the star from collapsing
against the force of gravity, lose their battle, and the white dwarf begins to collapse. As
you may recall, white dwarf stars are not made of Iron, (instead they are composed of
Carbon and Oxygen) and there is still substantial amounts of nuclear energy left in their
atoms. As the white dwarf begins to collapse against the weight of gravity, this material
is ignited, and rather than collapsing further, this nuclear blast wave consumes the star in
a second, creating an explosion 10 to 100 times brighter than a Type II supernova.
SN 1994D observed with the
Hubble Space Telescope. The SN is the bright star in the lower left corner.
Type Ia supernovae in the nearby universe are observed to all have a similar brightness,
and this makes them very powerful objects for measuring distances. In addition, because
they are so bright, they can be seen at great distances, and these two things make them
currently unique objects for measuring the vast distances of the Universe. Unfortunately,
they are very rare. The last one seen in our galaxy was in 1006, and it must have been
incredibly bright - easily visible in the daytime.
The idea to measure the Universe with Supernovae is not new, it has long been
contemplated, but it is only in the past decade that it has become feasible. The first distant
SN Ia was discovered in 1988 by a Danish team, but it wasn't until 1994 that they were
discovered in large numbers. Since 1995 two teams have been discovering these objects:
Our team, the High-Z SN Search, and the Supernova Cosmology Project.
To measure the fate of the Universe, we need both distant and nearby
objects, as it is only through the comparison of nearby and distant objects that the
Universe's behaviour uncovered. Amazingly enough, the first good nearby sample was
only completed in 1996 by a group at Cerro Tololo Inter-American Observatory (CTIO),
and these objects are what enable us to use supernovae to measure the ultimate fate of the
Universe.
Type Ia Supernovae are not all exactly the same brightness. They vary by as much as a
factor of two. But Mark Phillips, Mario Hamuy and collaborators at Cerro Tololo Inter-
American Observatory in Chile showed that faint supernovae rise and fall very quickly,
whereas bright supernova brighten and fade much more slowly, By looking at how much
the objects faded in the first 15 days following maximum light, their work showed that
type Ia supernovae can give distances which are good to about 7% - equal to the best of
astronomical distance indicators.
|